Introduction of Molecule and Lattice
Molecules and lattices are two fundamental concepts in chemistry and materials science that describe the arrangement and structure of particles within various types of substances.An understanding of these differences between molecules and lattices is vital to comprehending the properties and behaviors of various chemical compounds and crystalline solids.Molecules are discrete entities composed of two or more atoms bound together into molecules. As the building blocks for chemical compounds, molecules play a pivotal role in defining both their physical and chemical properties.
Molecules range from diatomic gases like oxygen (O2) to complex organic molecules found within living organisms.On the other hand, lattices refer to the regular and repetitive arrangement of atoms, ions, or molecules within solid materials in an ordered and regular pattern.Lattices form three-dimensional frameworks of crystalline substances and account for their distinctive properties; they can also be divided into different types based on their symmetry or arrangement of lattice points.
Composition, structure, properties, and key differences of molecules and lattices. By engaging with these concepts we will gain an increased knowledge of how particle arrangements on both an atomic and molecular level affect behaviors and characteristics of various substances.Furthermore, examples and applications of molecules and lattices will also be examined from everyday life, industry, and scientific research settings.
Definition of a molecule
Molecules are chemically bound structures composed of two or more atoms connected by bonds. As the basic unit of any compound, molecules retain all their original chemical properties despite being electrically neutral entities.
Composed either entirely from one element (e.g. O2), or composed from multiple ones such as water (H2O), their particular arrangement and type of bonds between atoms determine their structure and properties – including whether or not they exist as discrete entities within larger structures, as well as playing key roles in chemical reactions, biological processes, material properties etc.
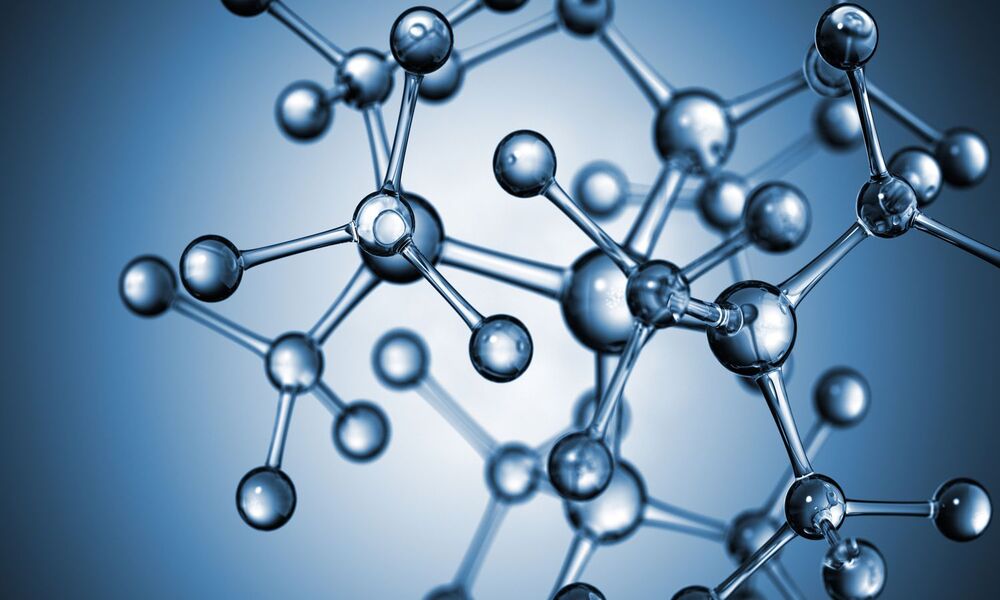
Definition of a lattice
Lattices are regularly and consistently repeating arrangements of atoms, ions, or molecules within solid materials that form a three-dimensional framework or structure of crystallinity.Lattice points represent each particle that occupies a particular spot within the lattice structure – with this being called its unit cell that characterizes it completely.
Lattices can be divided into various classes based on their symmetry and arrangement of lattice points, including cubic, tetragonal, orthorhombic hexagonal and rhombohedral lattice structures, among many others.Primitive designs contain only one lattice point per unit cell while non-primitive designs may feature several per cell.
Lattice structures are composed of interatomic or intermolecular forces such as ionic, metallic or covalent interactions that regulate their arrangement of particles in a lattice.Lattices determine physical properties such as density, melting point, hardness and optical properties in materials science, solid-state physics and chemistry fields – and therefore play a key role in defining macroscopic properties and behaviors of solid materials.
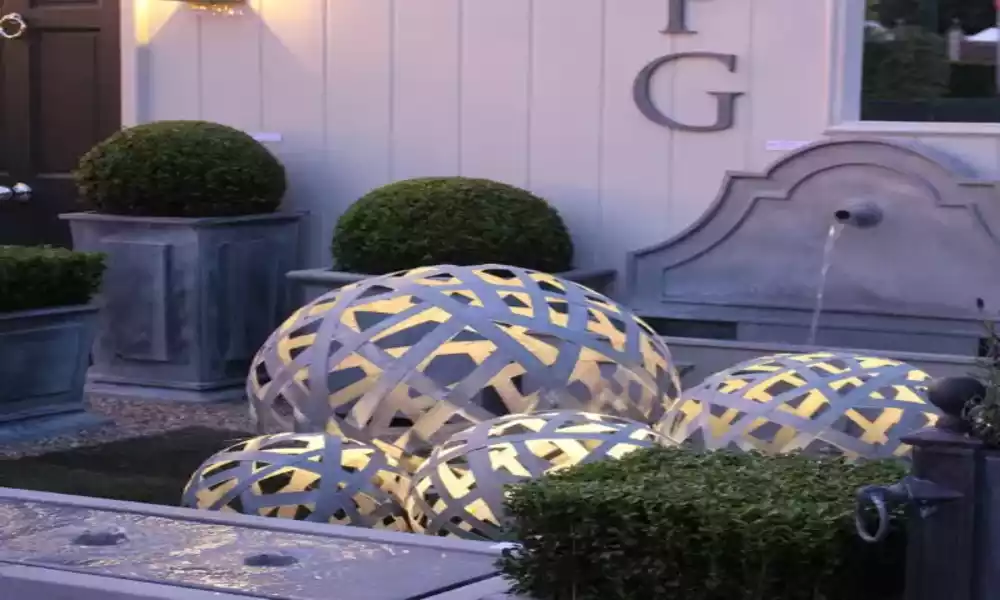
Comparison Table of Molecule and Lattice
Sure! Here’s a comparison table highlighting the key differences between molecules and lattices:
Aspect | Molecule | Lattice |
---|---|---|
Definition | A distinct entity formed by bonded | A regular arrangement of atoms, ions, |
atoms, consisting of two or more | or molecules in a crystalline solid | |
atoms | material | |
Composition | Composed of atoms or ions chemically | Composed of atoms, ions, or molecules |
bonded together | arranged in a periodic pattern | |
Bonding | Covalent or polar covalent bonding | Ionic, metallic, or covalent network |
between atoms | bonding | |
Physical | Lower melting and boiling points | Higher melting and boiling points |
Properties | ||
Solubility | Often soluble in polar solvents | Often insoluble |
Reactivity | Can undergo chemical reactions and | Generally exhibit limited chemical |
participate as discrete entities | reactivity due to tightly bound structure | |
Examples | Water (H2O), oxygen (O2) | Sodium chloride (NaCl), diamond (C) |
Please note that this is a simplified comparison table, and there can be variations and exceptions within each category. The properties and behavior of molecules and lattices can vary depending on specific compounds and materials.
Molecules have lower melting and boiling points
Molecules typically exhibit weaker intermolecular forces or bonds than larger molecules, leading to lower boiling and melting points. Such intermolecular forces include London dispersion forces, dipole-dipole interactions and hydrogen bonding – but these tend to be less strong compared with their internal strong bonds.
Lattices on the other hand have stronger interatomic or intermolecular forces holding particles together, leading to higher boiling and melting points. Their regular and repetitive arrangement enables stronger interactions among particles, necessitating more energy to dismantle it.
Lattices have higher melting and boiling points
lattice structures tend to have higher melting and boiling points compared to molecules due to stronger interatomic or intermolecular forces keeping particles together in its lattice structure.Lattices feature highly ordered and repetitive arrangements of particles, producing a strong cohesive force between constituent particles. This may take the form of ionic, metallic, or covalent network bonding forces which tend to be stronger than intermolecular forces in molecules.
Breaking a lattice structure and turning solid particles into liquid or gas requires significant energy; due to strong interparticle forces, lattices have higher melting and boiling points compared with molecules due to weaker intermolecular forces.Note that melting and boiling points vary based on the lattice type and intermolecular forces at work; different lattices have different bonding strengths which will affect their melting/boiling points.
Composition and structure
Composition and structure differ for molecules and lattices. Here is an overview of their composition and structure:
Molecules:
Composition: Molecules are composed of multiple atoms chemically bound together; these may or may not all belong to one element.
Structure of Molecules: Molecules have distinct structures. Atoms within a molecule are held together by covalent or polar covalent bonds which share electrons to create stable
molecular entities: Their arrangement within a molecule depends on specific bonding patterns and electron distribution.
Lattices:
Composition: Lattices are structures comprised of atoms, ions or molecules arranged in a regular and repeating pattern to form lattices. These particles may belong to either one type (such as elemental lattices) or multiple (such as in ionic or molecular lattices).
Structure: Lattices feature an extended and repetitive structure, wherein their constituent particles are arranged in three-dimensional form to form a crystal lattice.This lattice pattern can be represented by unit cells; their repeating nature gives an indication of its patterning; while particle placement within lattices is determined by their symmetric and periodic arrangement in relation to this crystal lattice structure.
Summary: molecules are composed of bonded atoms with discrete structures; lattices consist of particles arranged repetitively within an extended structure. While molecules feature covalent or polar covalent bonds that determine their configuration, lattices feature regular arrangements of particles within their crystal lattice that form an extended structure.
Atoms and chemical bonds
Atoms and chemical bonds play a pivotal role in the composition and structure of both molecules and lattices, providing essential support.
Here’s an explanation of atoms and chemical bonds as they pertain to molecules and lattices:
Atoms:
Atoms are the smallest unit of matter that maintain the chemical properties of an element, consisting of a nucleus containing protons and neutrons surrounded by electrons in energy levels or orbitals.Atoms combine together to form molecules or lattices. With molecules, atoms are bound together with chemical bonds to form an entity with different properties than its constituent atoms.
Lattices consist of crystal structures in which individual atoms (or ions or molecules) occupy specific positions within their crystal lattice structures, contributing to overall stability and properties of materials.
Chemical Bonds:
Chemical bonds are interactions among atoms which hold them together within molecules or lattice structures.
Molecules contain atoms bonded together via covalent or polar covalent bonds, with covalent bonds consisting of electron pair exchange between atoms that results in stable molecules.
Bonding within lattices varies according to the nature of particles involved, from ionic bonds between positively and negatively charged ions forming an ionic lattice to metallic bonding where electrons delocalized between metal atoms create a shared electron cloud; and covalent network bonding which occurs when multiple atoms are connected by an extended network of covalent bonds such as seen in materials like diamond or quartz.
Chemical bonds play an essential role in maintaining the stability, physical properties and reactivity of molecules and lattices. Their type and strength determine properties that influence behaviour across numerous chemical and physical processes.
Atoms are the fundamental units of matter and their interactions through chemical bonds define its composition, structure, and properties of molecules and lattices.Molecules form by bonding atoms together through covalent or polar covalent interactions while lattice structures may involve different kinds of bonds involving ionic, metallic, or covalent network interactions depending on the nature of particles in its lattice structure.
Properties of molecules
Properties of molecules encompass many characteristics that characterize their behavior, reactivity, and physical attributes.
Here are some key properties of molecules:
Molecular Mass: A molecular mass is defined as the sum of all of its constituent atoms’ masses; this provides information about both size and weight of molecules.
Molecular Formula: A molecular formula represents the type and number of atoms present in a molecule and provides a concise chemical notation of its composition.
Physical State: Molecules can take different physical states depending on the strength of intermolecular forces and environmental temperature and pressure conditions, including solid, liquid or gas states.
Melting Point: The melting point is the temperature at which solid substances transform to liquid state, determined by intermolecular forces within molecules.
Boiling Point: The boiling point is the temperature at which liquid substances change to gaseous form, determined by intermolecular forces and vapor pressure of molecules in solution.
Solubility: Solubility refers to the ability of a molecule to dissolve in a solvent and depends on intermolecular forces between solute and solvent molecules.
Density: Density refers to the mass per unit volume of any substance, providing information about how tightly packed molecules are within an area sample.
Polarity: Polarity refers to the distribution of electrical charge within molecules. Polar molecules possess an uneven distribution of charges that produce partial positive and partial negative charges; nonpolar molecules exhibit even distribution.
Chemical Reactivity: Molecules can participate in numerous chemical reactions, including synthesis, decomposition, oxidation reduction and acid-base reactions. Their reactivity depends on their chemical structure as well as the presence of functional groups.
Spectroscopic Properties: Molecules exhibit specific wavelength absorption, emission or scattering properties which can be analyzed using spectroscopic techniques and provide valuable insight into molecular structure and bonding relationships. These properties give information about molecular composition.These properties help define and understand the behavior and characteristics of molecules during chemical reactions, physical processes, or biological systems.
Molecular mass and formula
Molecular mass and formula are key properties of molecules that provide information about their composition and relative sizes.
Here’s a deeper dive into molecular mass and formula:
Molecular Mass:
Molar mass, also referred to as molecular weight, is defined as the total of all the atomic masses contained within a molecule. To calculate it, simply add up all the individual atomic masses within that molecule; typically expressed either in terms of atomic mass units (u) or grams per mole (g/mol).Molecular mass is used to give an idea of the size and weight of molecules. This information can be useful in various calculations, such as calculating moles of substance or reaction stoichiometry.
Molecular Formula: Whilst molecular formulae don’t reveal all aspects of an element’s composition, they provide a useful way of notating their chemical makeup quickly and concisely.
Molecular structure can often be represented using the elements with subscripts to indicate how many atoms each element contributes to its constituent molecule.
A molecular formula gives us an indication of the relative proportions between different atoms in a molecule.
For instance, water has the formula H2O to indicate its composition: two hydrogen and one oxygen atoms.Molecular formulae provide insight into the connectivity and structure of molecules, aiding in distinguishing among isomers or compounds with similar elemental compositions.
Molecular mass and molecular formula are intimately intertwined.
While the former specifies the number and types of atoms present in a molecule, its molecular mass quantifies their total mass in terms of atomic mass units or grams per mole. Calculating molecular mass using its constituent atom’s masses from its molecular formula provides another means of estimation.Molecular mass and formula play an essential role in identifying molecules, understanding their properties and anticipating how they’ll behave in chemical or physical processes.
Physical properties
Physical properties of molecules refer to traits that can be observed or measured without altering their chemical makeup, providing insight into how molecules interact with their environments and respond under various conditions.
Here are some commonly encountered physical properties:
State of Matter: Molecules can exist in different states of matter – solid, liquid and gas – depending on factors like temperature and pressure. Each form possesses specific physical properties like shape, volume and compressibility which influence their behavior.
Density: Density refers to the mass/volume ratio of any substance and provides insight into how tightly its molecules are packed together within its sample. Density can fluctuate with temperature and pressure changes.
Melting Point: The melting point is the temperature at which solid substances transition into liquid states, marking their transition from an ordered and dense arrangement of molecules into more disorganized configurations.
Boiling Point: The boiling point is the temperature at which liquid substances transition to gaseous states, signaling their transformation from liquid into vapour phase and being determined by intermolecular forces within their substances.
Solubility: Solubility refers to a substance’s ability to dissolve in a specific solvent, and depends on both its molecular makeup and interactions between solute molecules and solvent molecules.
Vapor Pressure: Vapor pressure refers to the force exerted by a substance’s gaseous phase in equilibrium with its liquid or solid phases, reflecting their tendency for molecules to move from solid states into gas phase states.
Electrical Conductivity: Electrical conductivity measures the ability of a substance to conduct electric current, with this factor depending on various factors like its presence of charged particles (ions) or electron mobility within its molecules.
Refractive Index: Refractive index measures how light bends when passing through materials, depending on molecular structure and light speed within that medium.
Optically Active Substances: Optically active substances have the power to alter the plane of polarized light by virtue of chiral molecules which lack symmetric symmetry, leading to optical activity and thus creating optical activity.
Thermal Conductivity: Thermal Conductivity measures the ability of Materials to conduct heat. It depends on factors like their Molecular structure, density and Intermolecular Interactions.
Physical properties of Molecules are crucial in Understanding and predicting their behavior across many physical processes, such as phase Transitions, solubility and thermal Conductivity.Furthermore, these Characteristics have practical Applications across a range of fields including materials science, Pharmaceuticals and Environmental science.
Crystallographic planes and directions
Crystallographic planes and directions are key concepts in crystallography – the study of crystal structures. They refer to specific orientations or arrangements of atoms or lattice points within a crystal lattice.
Here’s an explanation of crystallographic planes and directions:
Crystallographic Planes: Crystallographic planes are imaginary surfaces or planes that connect lattice points within a crystal lattice. Their position within this lattice can be determined using Miller Indices; three integers representing where these planes intersect with crystallographic axes.
Miller Indices can be calculated by multiplying the reciprocals of intercepts with a common factor to get integer values. Crystallographic planes are designated using square brackets such as (hkl), to indicate their specific orientation within the crystal lattice.Different crystallographic planes possess distinct properties, including surface reactivity, cleavage and lattice spacing which can be assessed through experimental techniques like X-ray diffraction.
WDG Directions of Crystallographic Planes (WCDGs):
Crystallographic directions are imaginary lines or directions which correspond with specific atomic rows, columns or lattice vectors within a crystal lattice.Direction indices, represented by three integers (uvw), represent ratios between lengths along crystallographic axes.
They can also be determined by taking reciprocals and multiplying them with a common factor to arrive at integer values.Crystallographic directions are indicated with angle brackets to denote their precise orientation within a crystal lattice.Different crystallographic directions possess unique properties, such as their density of lattice points along their respective directions and spacing between atomic planes perpendicular to these directions.
Crystallographic planes and directions play an essential role in understanding the symmetry, morphology and physical properties of crystals.They provide a systematic way of describing the arrangement of atoms or lattice points within a crystal lattice as well as aiding studies related to crystal growth, crystallographic defects and texture studies.Crystallographic planes and directions have wide application across disciplines such as materials science, mineralogy, solid state physics as well as crystallography itself.
Examples and Applications
Crystallographic planes and directions have many uses in different fields.
Below are a few notable applications:
Crystal Structure Determination: Crystallographic planes and directions play an essential role in identifying materials’ atomic arrangement and crystal structures.
Techniques like X-ray crystallography and electron diffraction use this data from crystallographic planes and directions to analyze diffraction patterns produced by them to precisely pinpoint positions of atoms within crystal lattice structures.
Materials Science and Engineering: Crystallographic planes and directions play a pivotal role in understanding and engineering material properties.
By shifting crystallographic planes and directions to control material properties such as mechanical strength, electrical conductivity, optical behavior or optical clarity – it becomes possible to manipulate properties like mechanical strength, conductivity or optical behavior to tailor materials specifically to applications that demand them.This knowledge helps material scientists optimize materials for specific uses.
Crystal Growth and Epitaxy: Crystallographic planes and directions influence both crystal growth and epitaxial alignment. By controlling their orientation during crystal growth, single crystal materials with desired properties can be produced.Epitaxial growth – where crystals are grown on substrates with matching crystallographic orientation – is often employed in semiconductor manufacturing or thin film deposition applications.
Mineralogy and Geology: Crystallographic planes and directions can be used to classify and identify minerals based on their crystal structures. Each mineral exhibits unique crystallographic planes and directions characteristic of its composition and crystal system, making analyzing this data essential in both mineral identification and understanding geological processes.
Material Characterization: Crystallographic planes and directions can help characterize materials. Electron Backscatter Diffraction (EBSD) and Scanning Electron Microscopy (SEM) techniques can map crystallographic orientations as well as evaluate grain boundaries, textures, and crystallographic defects present within materials.
Nanotechnology: Crystallographic planes and directions play an integral part in nanoscience and nanotechnology, as understanding their arrangement at the nanoscale allows engineers to engineer nanomaterials with desirable properties such as enhanced catalytic activity, optical behavior or mechanical strength.
Structural Biology: Crystallographic planes and directions play an essential role in studying biological macromolecules such as proteins and nucleic acids. X-ray crystallography allows scientists to map out their three-dimensional atomic structure, providing insight into their function and interactions.These examples and applications demonstrate the significance of crystallographic planes and directions in various scientific disciplines, providing greater insight into materials, minerals, biological molecules, and nanoscale phenomena.
Conclusion
Crystallographic planes and directions are fundamental concepts in crystallography, denoting specific orientations and arrangements of atoms or lattice points within a crystal lattice.Crystallographic planes are imaginary surfaces defined by Miller indices while crystallographic directions are imaginary lines defined by direction indices; both play an instrumental role in defining crystal structures, understanding material properties, guiding crystal growth control strategies, characterizing materials and creating databanks for scientific analysis.
Crystallographic planes and directions have many applications in various fields, such as materials science, engineering, mineralogy, geology, nanotechnology and structural biology.For instance, their study can aid in crystal structure determination; designing materials with desired properties; identifying minerals; studying nanoscale phenomena and predicting biological macromolecule structures.By manipulating crystallographic planes and directions, scientists and engineers can gain valuable insights into how atoms within crystals arrange and behave, leading to advancements in materials design, technology development, scientific understanding and more.